kHz multiphoton imaging
There have been several reports recently about ultrafast multiphoton imaging.
To start, here is a brief review of the conventional ways to get ms-level resolution:
(1) Cycle through fewer pixels. E.g., it is trivial to line scan a dendrite and get ms time resolution, and people have been publishing data like that for decades. Here’s an example from 1997 of imaging dendritic calcium transients in vivo in rats at 0.5 kHz. Of course, the tradeoff is pixel count for time resolution.
(2) Use a resonant scan mirror. These can provide approximately 4x line scan rates. Combined with strategy 1 above, ms-level resolution can be obtained for even larger line count frames. They have some drawbacks (e.g., no arbitrary control– just on/off and scan magnitude, no offset), but are relatively straightforward to implement.
(3) Use acousto-optical deflectors (AODs). These scanning devices are fast and can be used in an array of different configurations for different scan types. They can be used to rapidly scan or jump from location to location in 3D or other configurations. Saggau, Helmchen, Rózsa, Konnerth, Silver, and Dieudonné are among the labs that have developed novel implementations of AOD scanning for in vivo multiphoton use. Like all technology, they have tradeoffs. They’re dispersive, so compensation is necessary. Scan angles are small, so fields of view in XY are typically modest. Apertures are also small, because as the size is scaled up, the scan speed decreases. So scaling up to large back aperture objectives (as used in large field-of-view systems) is not typically done. Still, they have a niche, and work great in special applications.
The recent papers discussed below each provide creative new approaches for obtaining ms-resolution scanning. Let us compare and contrast them a bit.
Reverberation / ring-down / multiple reflection – type approaches
These are elegant approaches that use simple reflections to multiply the pulses either laterally (X) or axially (Z) in space.
The Z-version of this just appeared in Nature Methods. It’s work from Jerome Mertz’ lab. It involves a reverberation loop where the pulses pass through a lens on each trip through the loop. Each pass slightly changes the convergence of the light, and thus shifts the focal plane slightly. The pulse also loses power on each loop, and more power is needed deeper, so the least reverberated pulse is focused deepest. Temporal demultiplexing is then used to separate the layers back out. The practical limit appears to be about 5 planes, but if one wanted to combine this approach with computational unmixing of multiple layers (e.g., constrained nonnegative matrix factorization) then a factor of up to 4 (e.g., 20 layers total) is feasible, depending on the preparation. Maybe more.
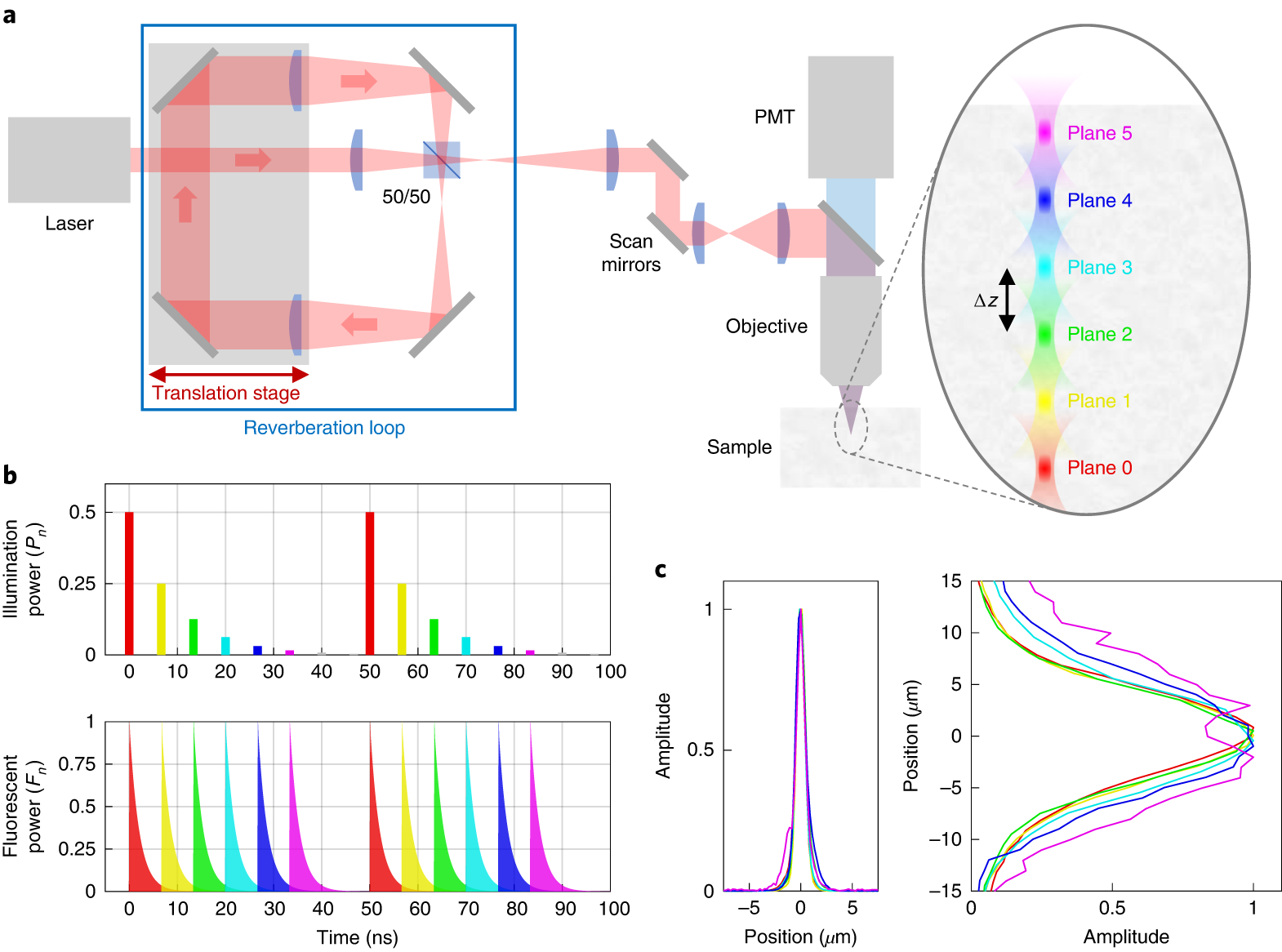
The X-version of this was recently published as a preprint from Na Ji’s lab, building on collaborator Kevin Tsia’s 2017 work. Peter Rupprecht noticed Kevin’s paper when it came out and discussed its potential, ending his piece with, ” Certainly a lot of work is required to transition this from an optical table to a biologist’s microscope, but I hope that somebody accepts this challenge…”. Luckily for all of us, an excellent scientist, Na Ji, did just that! Like the Z version above, it is difficult to scale up the number of scan points. That is, the number of pixels on the fast axis is limited. In this work, they got 80 beamlets into a 50 micron wide stripe, and obtained data at 1000 fps. Voltage imaging in dendrites could be a good application.
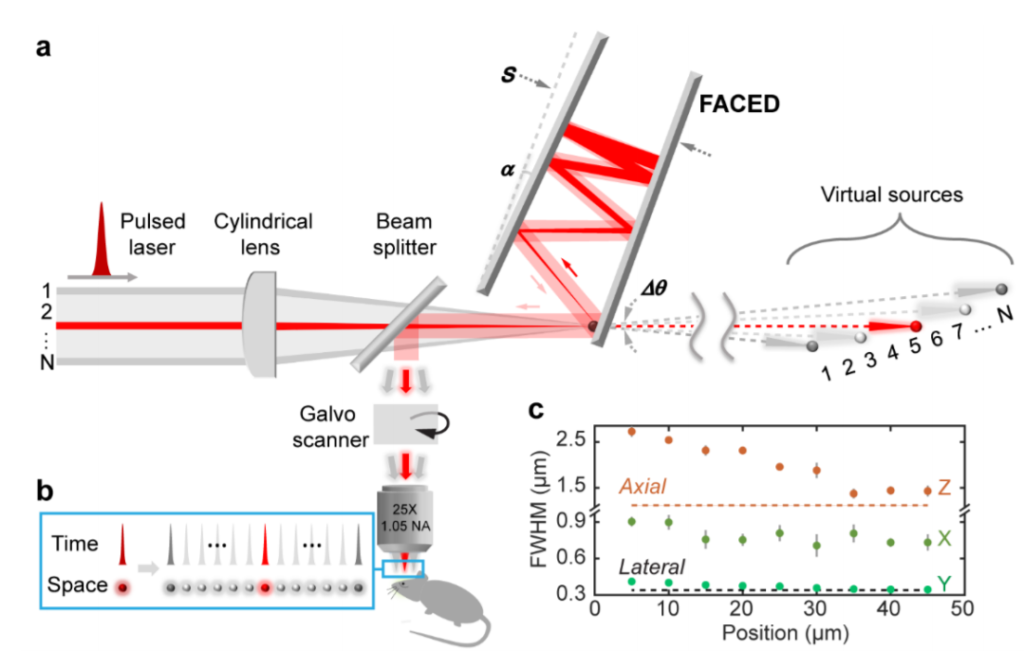
Computational
Kaspar Podgorski developed a tomography-based system for rapid imaging. This approach is computation heavy, and is ideal for relatively sparse samples– many neuroscience applications fit in this class, including input mapping.
All other approaches in discussed here are point scanning approaches, where raster scanned or random access. Instead, Kaspar’s approach uses lines of excitation. When lines are scanned at different angles (this is the tomography part) and structural information is considered (obtained initially), fast dynamics can be recovered computationally.
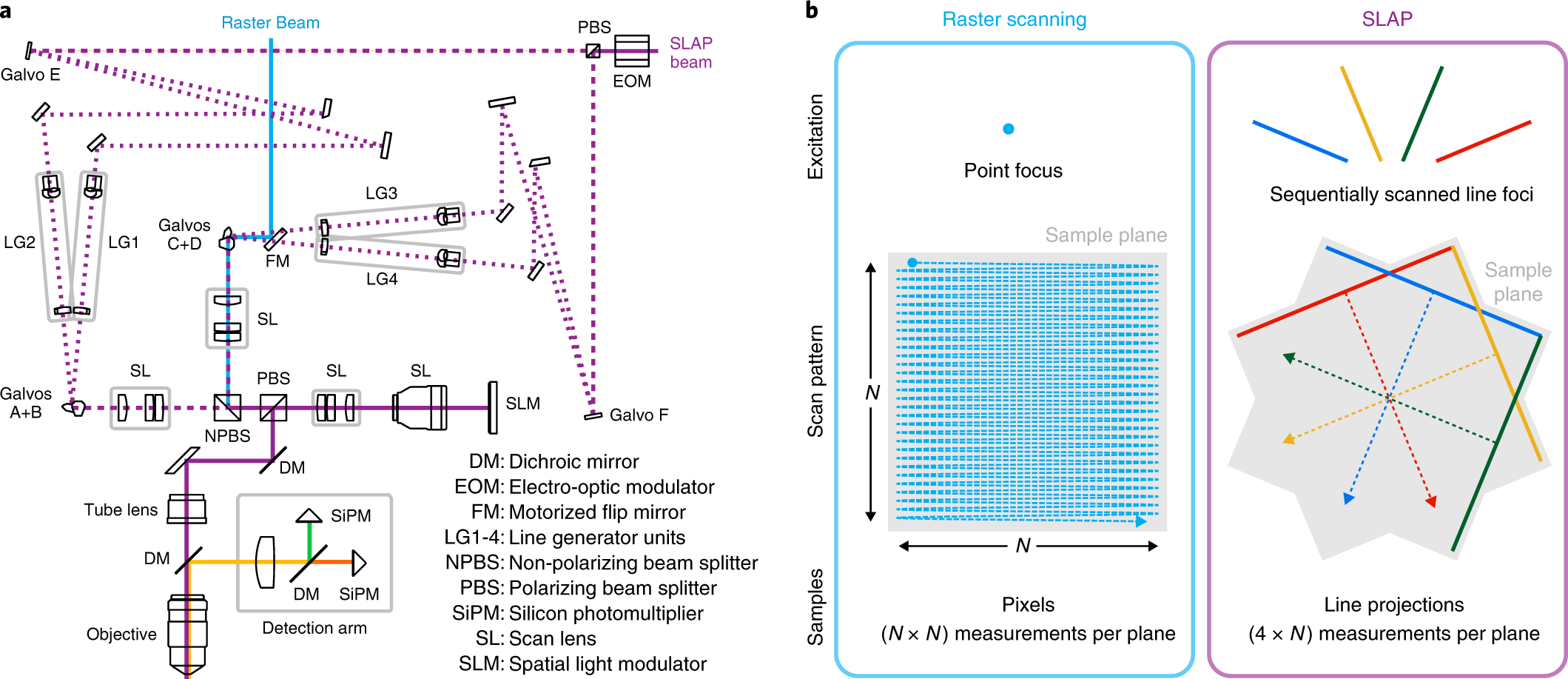
Multi-beam, multi-detector
A team from Mark Schnitzer’s lab used a multibeam approach with spatial demultiplexing, using a camera, to attain 1000 fps imaging rates. With enough spatial separation, they were able to obtain high fidelity data. The performance degrades as scattering increases (e.g., imaging deeper), naturally, but the paper provides some empirical data on that. For the right experiment, this could be very useful.
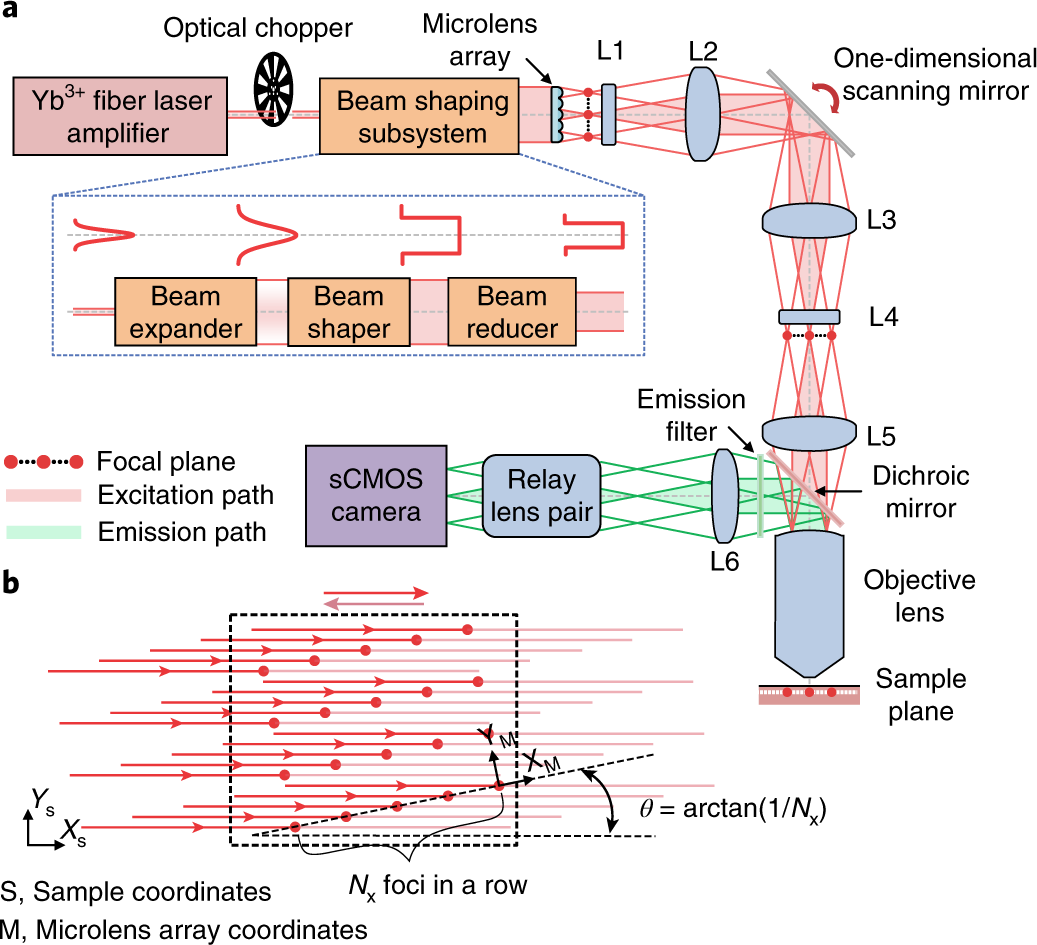
[…] To find alternative methods for upgrading scan rates, several recent methods papers used variations on “parallel scan” approaches. These authors divided the excitation beam into multiple beamlets to increase the scanned area. Fluorophores in the target volume of the sample were excited in quick succession (nanosecond scale), and processed with fast detection and acquisition electronics, thereby conserving the spatial resolution. In other instances, images were processed in parallel by using an array detector, using sCMOS or CCD cameras. All these methods compromised on some other aspects when compared to the performance of the AOD scanners. Nonetheless, many more developments are sure to follow. These methods were summarized well be Spencer LaVere Smith in a recent blog post. […]